Neurophotonics
Using light to enlighten the brain function
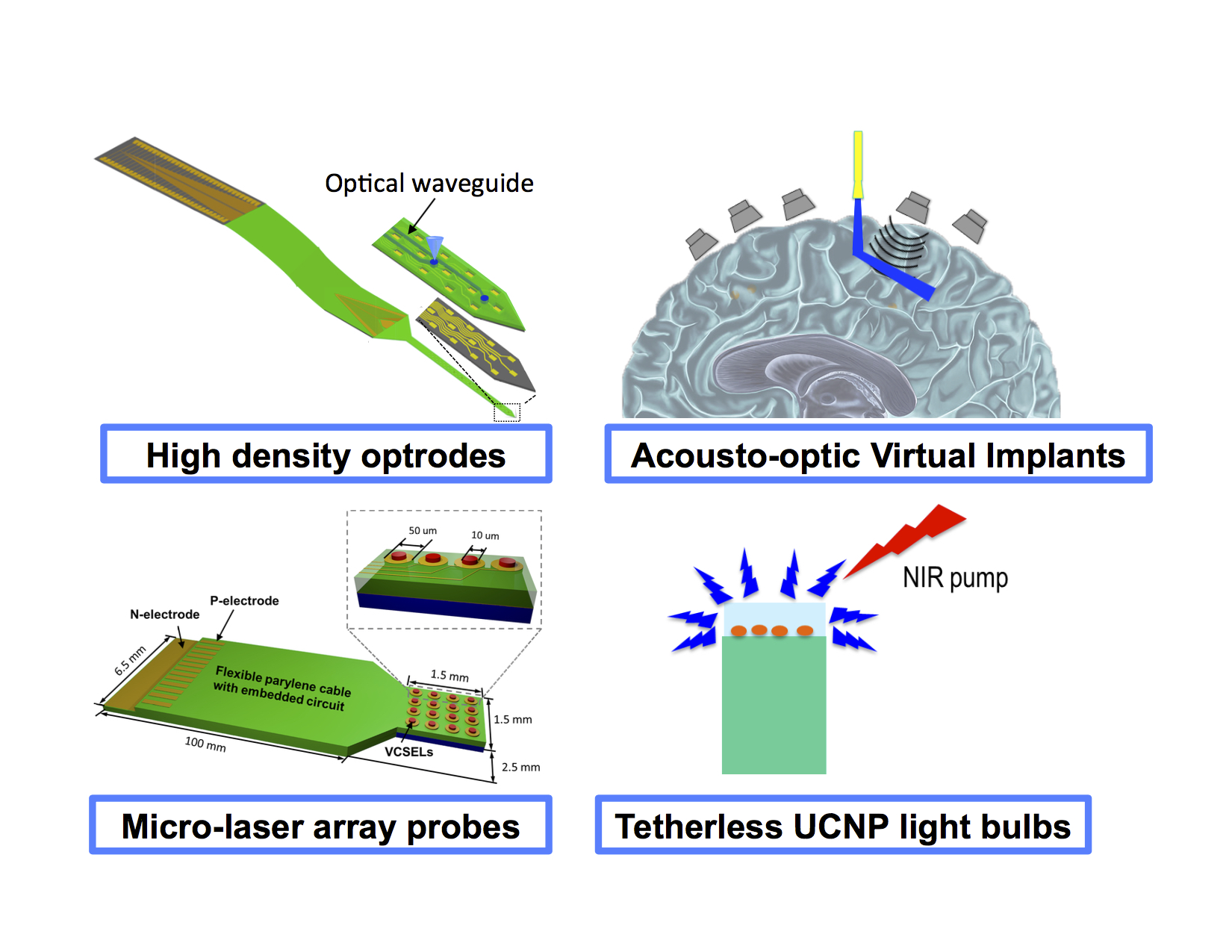
Understanding the neural basis of brain function
and harnessing its power is arguably a grand challenge of our era. Brain is a
very complex organ composed of billions of neurons that form circuits and
networks that mediate our behavior. Our research is focused on developing novel
tools and techniques to better understand the brain function. Having a clear
understanding of the application space in neuroscience and biophotonic, and a
deep knowledge of applied physics, we design, and build next generation neural
interfaces. Using our neural interfaces we target areas of application both in fundamental
neuroscience and Brain-machine interfaces (BMIs). Using concepts in electromagnetics, semiconductor physics, optics, MEMS, and material science
we are developing multimodal (acousto-opto-electrical) neural interfaces. The scope of research in our group encompasses
theoretical design and simulation, fabrication and packaging, experimental benchtop characterization, as well as in-vivo, in-vitro, and ex-vivo tests on
biological systems.
While the main focus of our current research is on neural engineering, we have a couple of active projects on photonics and nanoplasmonics. In particular, we are working on a novel technique to obtain the 3D functional map of retina in one snapshot for early detection of diabetic retinopathy before the onset of structural damage.
Virtual Acousto-optic Neural Implants
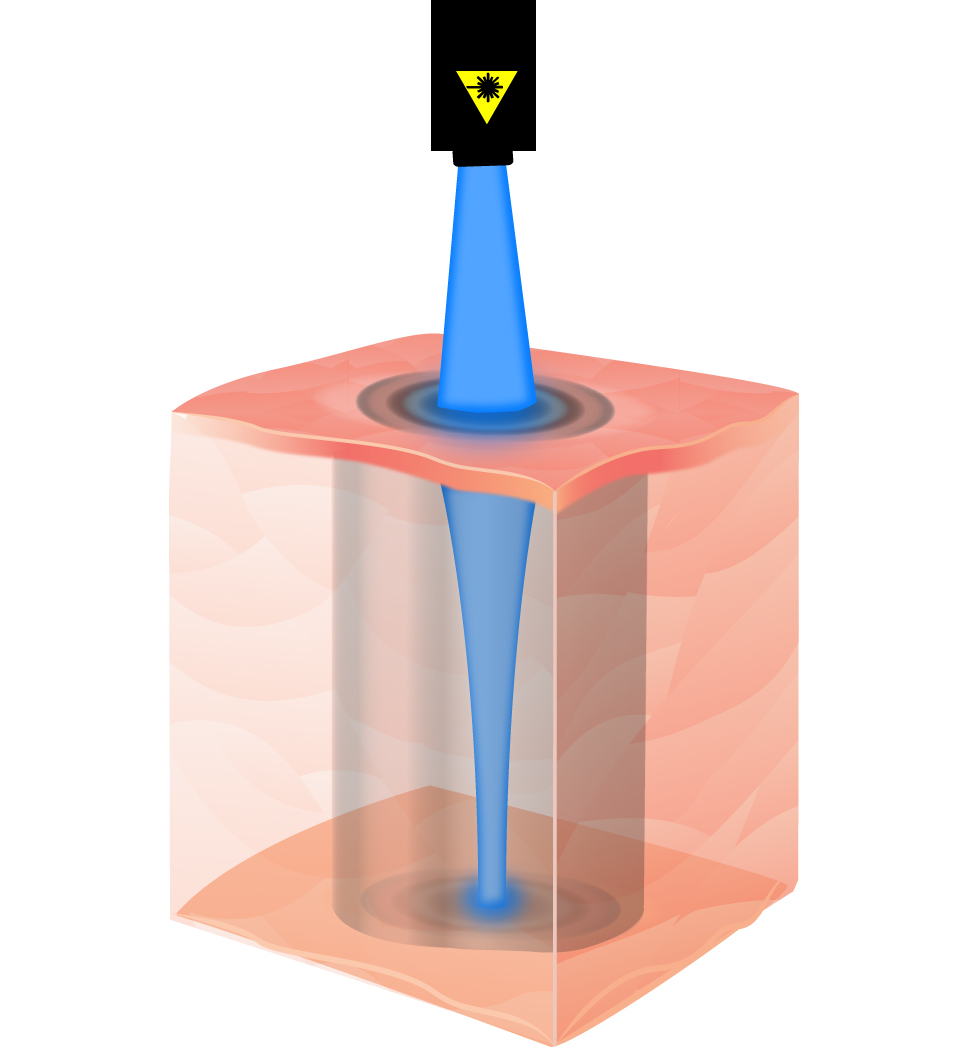
Optical imaging is the gold standard of non-invasive biological imaging. Moreover, optical stimulation techniques are rapidly expanding. For example, optogenetics has proven to be a powerful method for neural stimulation. However, two serious challenges need to be addressed for optical interrogation to be effective for deep-tissue imaging and stimulation. One is targeted delivery of light deep into the brain tissue (at least a few millimeters) and second is non-invasive beam steering in the tissue. Current methods of light delivery and beam steering require inserting optical fibers, waveguides, or active light sources, which would cause a significant tissue displacement and damage to the vasculature. In this project, we take a radical approach to use ultrasonic waves to define and steer the trajectory of light in the brain for non-invasive targeted optogenetic stimulation and imaging of local neuron clusters. We are applying this technique to selectively stimulate different basal ganglia nuclei to come up with new therapeutics for Parkinson's disease.
High Density Optrodes for Neural Recording and Stimulation
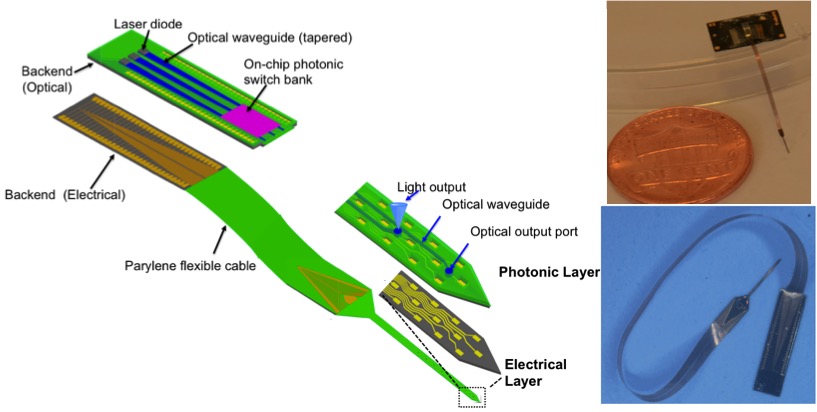
In this project, we are developing high-density, hybrid polymer-silicon, neural probes capable of recording from a large population of neurons with single ubit resolution. Our neural probes consist of 64 channel silicon shanks, monolithically integrated compliant flexible cables, and a stiff backend that can be integrated with the recording headstage circuitry. Our scalable fabrication process based on high resolution DUV lithography is optimized on different fronts to develop reliable neural probes. The manufacturing process and the post-fabrication assembly are optimized so that a large number of these neural probes can be made available to a wide user base. We are testing these probes for full-volume sampling of a mouse visual cortex in V1 and HVAs. We are now adding optical functionality through integrating on-chip photonic waveguides and optical switch banks. Using integrated photonic techniques, we design novel architectures for implantable neural probes based on biocompatible materials to realize higher density probes that can be actuated while in the brain.